Unpacking Renewable Intermittency: Challenges and Solutions for a Sustainable Energy Future.
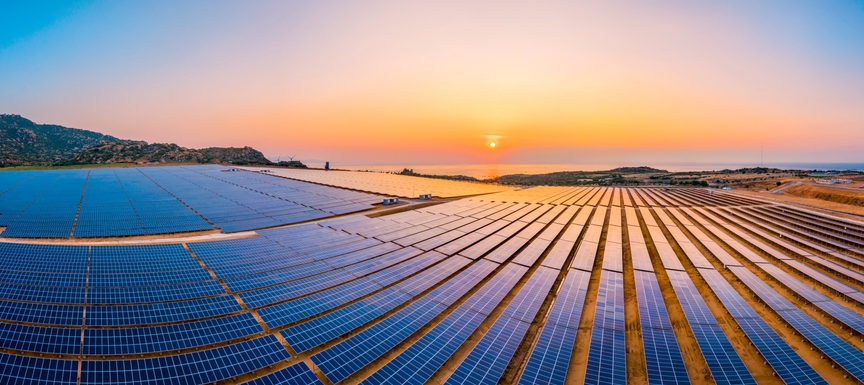
The path to carbon neutrality requires proliferation of renewable generation. Despite a significant increase in generation capacity, the intermittent nature of renewables constrains its ability to meet consumer demand, particularly at the time when it is needed most. Furthermore, desirable geographic locations for renewable generation are incongruous with legacy transmission infrastructure designed for fossil fuels.
Given these constraints, how can we unlock the true potential of renewables?
The task at hand
As part of its Net Zero Roadmap for the Global Energy Sector, The International Energy Agency has modelled a four-fold increase in renewable capacity from 2020 to 2030, with Variable Renewable Energy (VRE), i.e., solar and wind, forecast to generate over 60% of the world’s electricity by 2050, from less than 10% today1. No matter which way you cut it, VRE will play a critical role in the energy mix as countries execute their energy transition plans.
There are significant tailwinds to boost renewable generation capacity. Solar PV and onshore wind are now cheaper than coal-fired power stations and natural gas2, and governments are investing heavily to add VRE capacity and supporting infrastructure.
However, despite the environmental and economic advantages of VRE, achieving IEA targets will be difficult. VRE is intermittent in nature – on land, the sun doesn’t shine 24/7, and wind speeds are variable, which poses two key challenges for industry participants.
Timing and transmission: the dual challenge of intermittency
Firstly, VRE supply is typically high during periods where consumer demand is relatively low. Take solar PV for example - irradiance (the amount of light energy hitting each solar panel) is typically highest around midday local time, which drives peak energy output for a solar PV generator. Unfortunately, consumer demand tends to be relatively low during the same period. Energy consumption levels in most countries peak in the mornings (between 07:00 – 10:00 local time) and evenings (between 18:00 – 21:00 local time).
Last week’s consumption in California illustrates the hourly changes in consumption each day. Overlay a typical generation profile for a utility-scale solar PV farm in California, and you can see the mismatch between peak supply and peak demand. In this example, solar PV doesn’t generate power during the top quartile of consumption hours3,4.
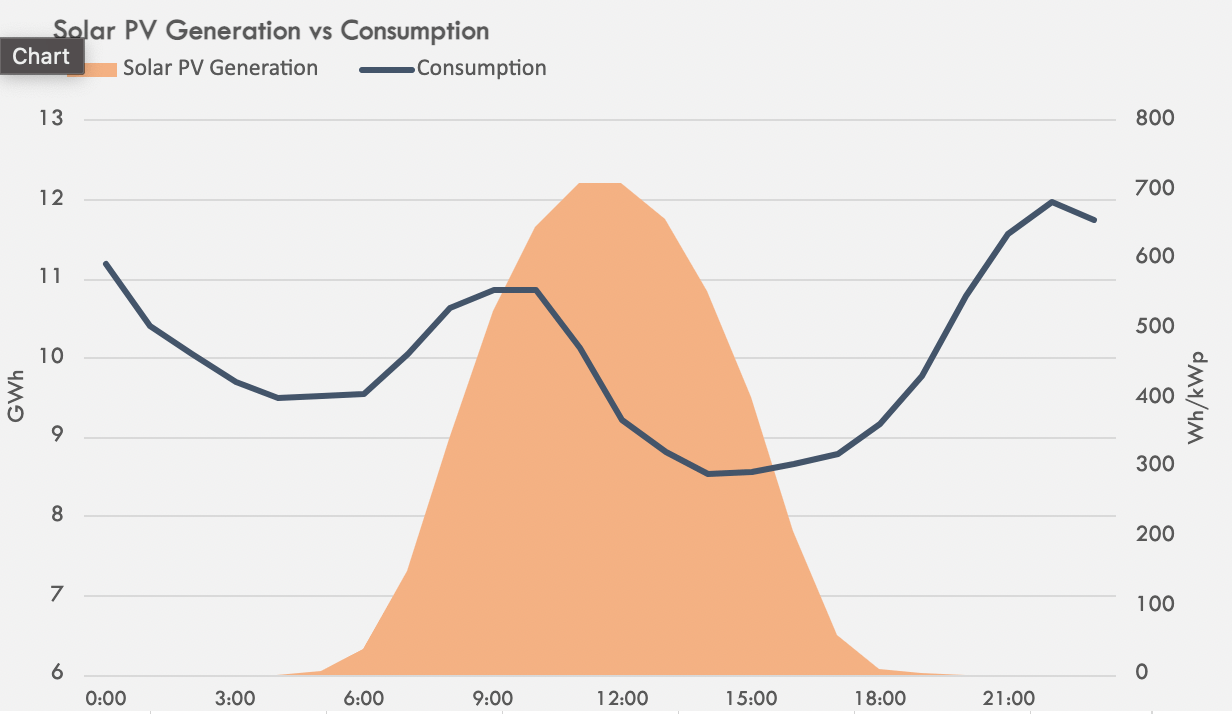
Secondly, renewable generation assets generally have higher power output potential in remote locations (e.g., deserts for solar and seas for wind) that are further away from high-capacity transmission infrastructure.
Grid infrastructure has existed for decades, and electricity transmission is over a century old. The first-long distance transmission of DC electricity in the United States was commissioned in 1889, at Willamette Falls Station in Oregan5.
Electricity grids were built close to population centres, as were the fossil fuel generators they connected to. Over decades, grid operators have continued to use the same philosophy, with high-capacity transmission lines connecting large fossil fuel generators close to large population centres.
Unfortunately, this decades-old system is far from optimal for VRE.
Consider Australia, a country with vast solar resources, the highest potential of which lies in the middle of the country. Currently, little transmission infrastructure exists to capitalise on Australia’s rich renewable generation potential in the centre of the country. Australia’s transmission infrastructure illustrates the challenge in connecting optimally placed renewables to the grid6,7.
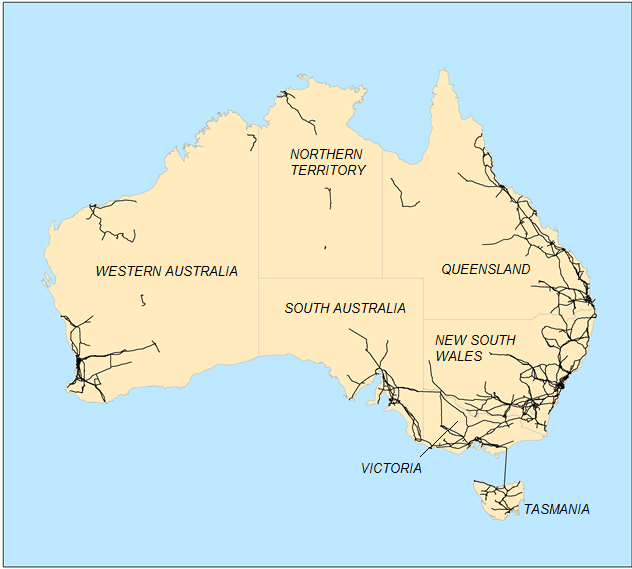
A related issue is the cost of land and difficultly in accessing land close to population centres. VRE developers can spend a significant amount of time sourcing and negotiating parcels of land to begin their projects.
The result of optimising the location of VRE assets at the expense of high-capacity grid connectivity is curtailment.
Curtailment: the consequences of intermittency and legacy transmission infrastructure
Curtailment, or more specifically, generation curtailment, refers to the reduction of power production of a power generating asset8. This typically occurs during peak VRE generation periods, primarily affecting generators further away from high-capacity transmission infrastructure.
This can partly explain why it is more likely that a grid operator will curtail a renewables generator as opposed to a fossil fuel generator. Curtailment can stunt the growth trajectory of renewables capacity.
How does this play out in real life? Consider South Australia.
The proportion of VRE generation in South Australia is amongst the highest in the world. In December last year, during summer in Australia, wind and solar contributed 85% of the state’s electricity demand9. Over the ten days between the 9th and 19th of December, average production of wind and solar covered 100% of demand9.
However, South Australian VRE assets experience significant curtailment. Last November, curtailment peaked at 1,307MW at 1:55pm, which represented 94% of native demand. In other words, VRE supply was nearly double demand. Over the course of the day, Australia’s National Energy Market (NEM) curtailed nearly 40GWh of VRE, the equivalent of 20% of native demand10.
As curtailment losses increase, VRE financiers may be less likely to offer competitive funding to developers who cannot sell their full generation profile. Generators may be forced to sell their generation into a spot market already saturated with renewables, reducing the economic viability of new projects.
Thankfully, several current and emerging technologies can help reduce curtailment by addressing the challenges of intermittency in a variety of innovative ways.
Time-shifting: aligning supply and demand.
Correcting the mismatch between peak generation and peak consumption requires time-shifting supply and/or demand.
Time-shifting solutions broadly fall into two buckets: storing excess generation capacity to deploy at a different time (time-shifting supply), and re-allocating energy consumption to match periods of peak generation (time-shifting demand).
Batteries are currently the predominant mechanism to time-shift supply on a short-term basis, with storage durations between a few minutes to four hours. For example, the Vistra Moss Landing Energy Storage Facility, the largest battery energy storage system in the world, has a storage duration of four hours (400MW/1,600MWh)11.
Green hydrogen may also play a role in longer-term storage, discharging during protracted weather events that may impact VRE generation over days and weeks12.
Time-shifting demand can occur by either increasing consumption during peak generation periods or decreasing consumption during periods of lower generation.
Moving non-time critical consumption next to renewable generating assets can provide local curtailment relief. For example, deploying non-time critical computing next to renewable generating assets, sucking up excess supply when grid operators instruct the generator to curtail.
Decreasing demand requires behavioural changes and smart consumption solutions. Occupancy sensors that ensure lights are turned off when no one is present, dynamic thermostat settings to reduce HVAC load during periods of peak demand, and incentivising timing of EV charging and home battery discharge are all tactical methods to time-shift demand13.
Transmission expansion: aligning generation and transmission locations.
Correcting the geographical mismatch requires deploying long distance, high-capacity transmission to connect VRE generators in optimal locations to end users.
Much effort is underway to increase the capacity of regional transmission networks to boost investment in renewable generation. In December last year, the Federal and New South Wales Governments of Australia entered into an agreement to provide a combined A$7.8 billion in financing to deliver eight transmission projects as part of the country’s Renewable Energy Zone initiative14. These zones provide higher capacity transmission when, coupled with more favourable regulation of grid access, can encourage development of VRE generation.
Feasibility studies are also underway to develop green hydrogen hubs in remote locations and subsea transmission infrastructure to connect neighbouring islands15.
Where’s does Emrod’s Worldwide Energy Matrix play its part?
Emrod’s Worldwide Energy Matrix (WEM) solves both time-shifting and transmission challenges at mega-scale, unlocking the next wave of renewables investment. Click here to view our article introducing WEM.
WEM effectively time-shifts supply by beaming power from locations of peak generation to locations of peak consumption. WEM moves power across tens-of-thousands of kilometres, unlocking a suite of new time-shifting possibilities.
For example, WEM can beam power from the United Arab Emirates (UAE) to Japan. Given the time differences between the two countries, daily irradiance levels in the UAE peak at precisely the time that Japan’s electricity consumption increases.
The benefits are clear for both countries. The UAE benefits from exporting its rich solar resource during peak generation periods, while Japan benefits from importing renewable energy at the time when it is needed the most.
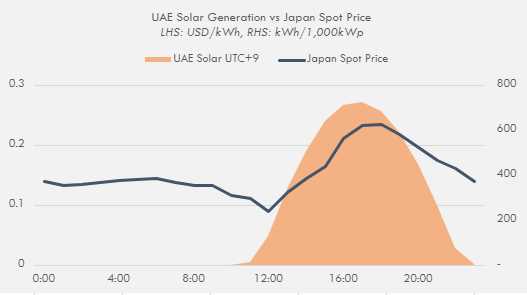
At scale, Japan can reduce its reliance on fossil fuel generation and defer capital investment in non-renewables generation, transmission, and VRE-firming technology, while UAE can boost its solar PV capacity to become a major exporter of renewable energy.
Nations can embark on mega-projects to add renewables capacity at scale. Such projects would become more attractive as the risk of curtailment is significantly reduced, and therefore, large-scale project financing may become more readily available.
WEM is a compelling addition to address the challenges of VRE intermittency and promote mega-scale development of VRE capacity across the globe.
Putting it all together
Despite the intermittent nature of VRE, a rich vein of innovation is addressing key challenges. From small-scale local solutions such as demand response, to national transmission strategies, to mega-scale regional solutions such as WEM, each solution will play an important role in boosting VRE capacity, displacing fossil fuels, and accelerating the transition to net zero.
Sources
1International Energy Agency (IEA), Net Zero by 2050 – A Roadmap for the Global Energy Sector, report
2International Renewable Energy Agency (IRENA) Renewable Power Generation Costs in 2021, report
3California Independent System Operator (CISO) energy consumption from 9th May 2023 – 15th May 2023 - https://www.eia.gov/electricity/gridmonitor/dashboard/electric_overview/balancing_authority/CISO
4Global Solar Atlas – snapshot of utility-scale solar PV power potential in selected location in California
5 "History of Station A". Willamette Falls Heritage Foundation. 2008. Archived from the original on July 16, 2012
6Global Solar Atlas – map of PV power potential in Australia
7Map of Australian transmission network, 2017. Geoscience Australia, The Australian Government
8https://www.next-kraftwerke.com/knowledge/curtailment-electricity
12IRENA Green Hydrogen Breakthrough 2021, report
15https://www.iea.org/data-and-statistics/data-product/hydrogen-projects-database